Basic Principles: Receive, Process and Export
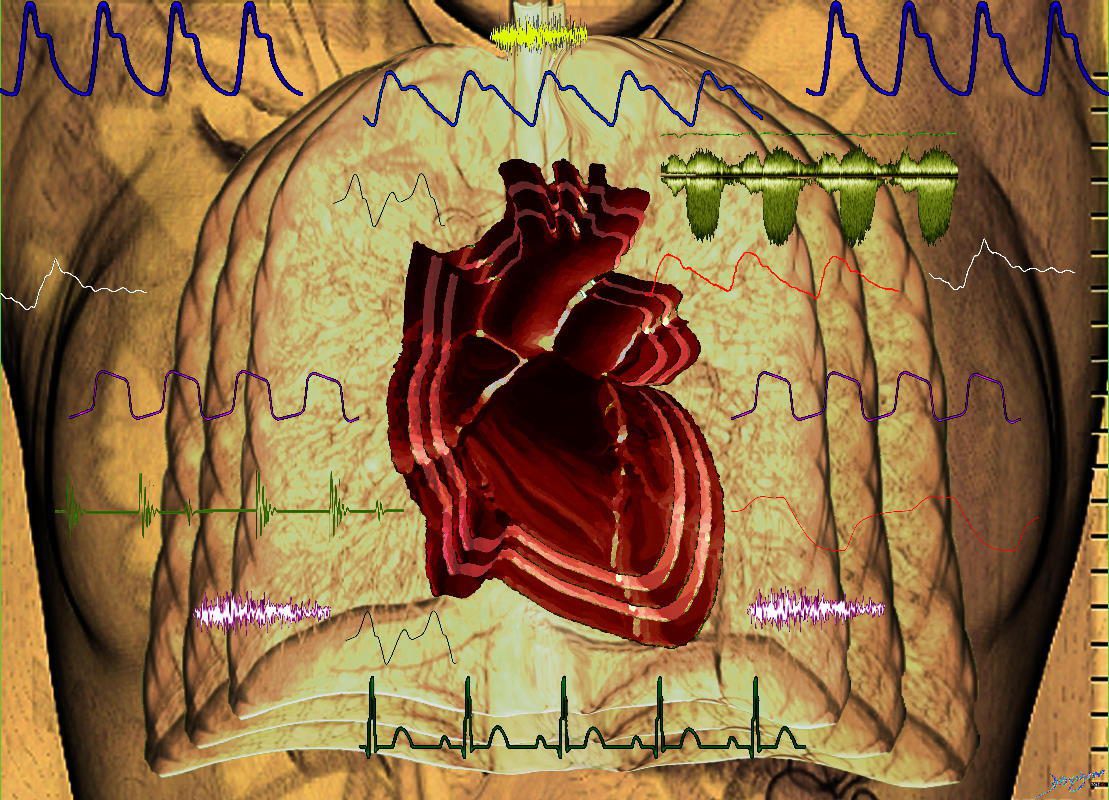
Ashley Davidoff MD TheCommonVein.net
heart-0112
The lung and airways have the important role of bringing fresh air from the environment to the alveoli, to enable a gradient for the oxygen and carbon dioxide to be exchanged, to facilitate the uptake of and removal of the gases from the blood stream and to transport the carbon dioxide to the environment.
In keeping with the basic principles outlined in the introduction, it receives the oxygen, transports it to the airways, processes by creating a filter and exchange mechanism, and then exports the oxygen intot the blood. It has a dual function in the it receives the carbon dioxide from the blood and then after assisting the the conversion of CO2 from a fluid envoronment to a gaseous environment, it moves the carbon dioxide to the environment.
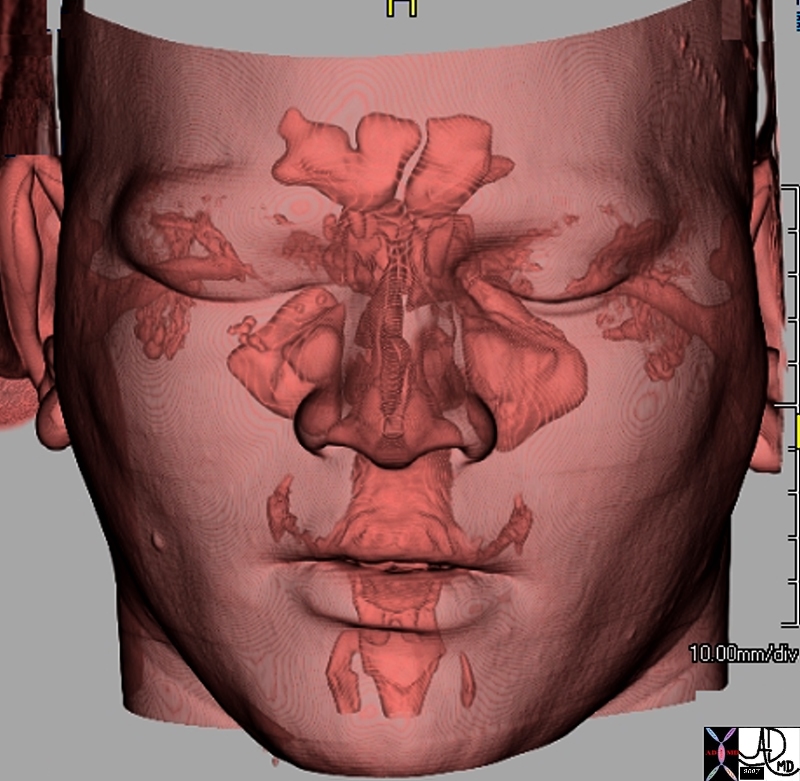
3D rendering of a CTscan of the head showing the sinuses including the frontal sinus, ethmoid sinus, maxillary sinuses, auditory canals, eustachian tubes, mastoid air cells, nasopharynx, oropharynx, nasal passages and sphenoid sinuses.
keywords # anatomy, normal, CTscan, 3D volume rendering,
Ashley Davidoff MD TheCommonVein.net 48728b01.800
Lung Movement : Basic Principles
The lung is often compared to a bellows system, meaning that with alternate expansion and contraction, air travels one way through a tube during expansion and expelled the other way when the mechanism recoils.
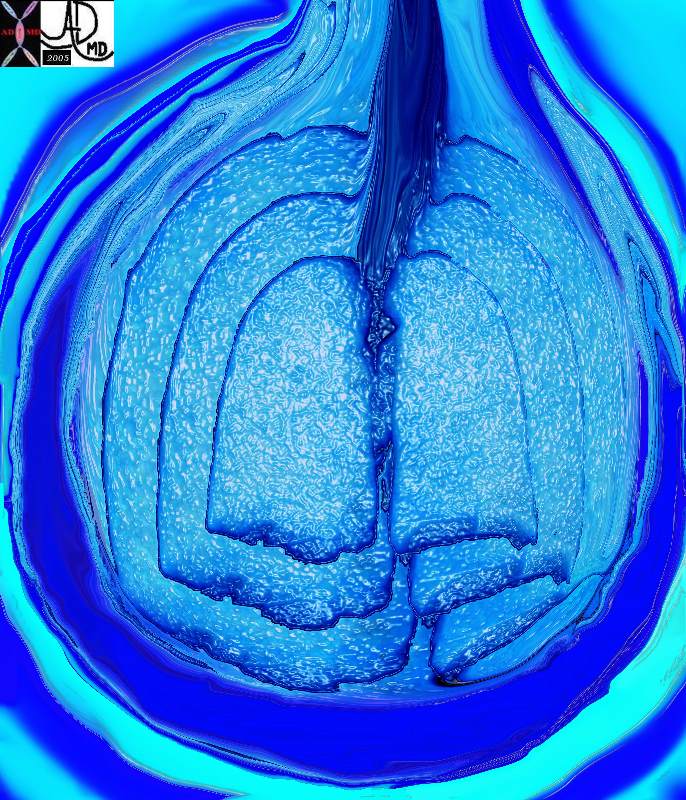
The chest quietly expands and contracts under basal conditions in order to serve the alveoli. At first glance it seems like a simple bellows-like process, but as one delves into the layers of detail, the complexity of the structural design unfolds as a combination of physical and chemical forces.
Ashley Davidoff MD TheCommonVein.net 42530b05b09b28
Inspiration is an active process requiring muscle contraction and therefore energy, while expiration is a passive process of elastic recoil requiring no energy. As noted, one of the unique features of the lung is its ability to act as a two-way transport system. No other tube in the body is asked to perform this dual function. At first this seems like a simple demand on the system, but as you will see it is more complicated than a simple bellows system, and a fine balance exists between the complex forces of inspiration and the complex forces of expiration. Biology has had to make some considerable adaptations in order for the dual function of the airways to coexist.
Lung Movement: 5 Layers
There are five general functional layers to the bellows system. They include the bony cage, the muscle layer, the pleural layer, the lung and the layer of surfactant. The overall complex with bony, muscular, membranous, spongy elastic and chemical properties keep the air moving in and out efficiently.
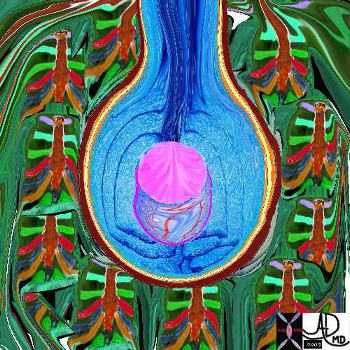
The five major layers that keep the air moving include the outer bony cage, the muscular layer represented in maroon, the pleural complex (orange yellow orange) the lung (blue) and surfactant within the alveolus. (pink)
42530b05b09b01a08
Ashley Davidoff MD
TheCommonVein.net
First Layer ? Bone
The bony cage of ribs sternum and spine create the outer protective layer with the sternum and spine being the relatively rigid components and the ribs being both protective and the more pliable component. The ribs move up and out during inspiration pivoting on the sternum and spine like bucket handles (ribs) on the two fixed points of the bucket (sternum and spine)

Courtesy of: Ashley Davidoff, M.D. TheCommonVein.net
42530b08
Second Layer ? Muscle
The second layer includes the diaphragm inferiorly, the intercostal muscles circumferentially, the serratus anterior anterolaterally, scapular elevators posteriorly and erector spinae muscles posteriorly. Superiorly the scalene and sternocleidomastoid muscles assist when necessary. The abdominal muscles also play a role as accessory muscles of respiration since movement of the chest cavity has effect on the abdomen, and vice versa. In fact, a principle to always keep in mind is that no cavity, system, organ or cell is an isolated island ? they all in the end work in concert for the greater health of the whole. John Donne, an English poet of the 17th century, proposed that ?no man is an island,? promoting the same concept of the interconnectedness of all.

The chest is surrounded by a ring of muscle (maroon) made up of a various groups which work in concert. The diaphragm is the workhorse of the respiratory muscles and is shown as a thick maroon band inferiorly.
Ashley Davidoff MD TheCommonVein.net 42530b05b09b14
During inspiration the diaphragm moves down and intercostal muscle contraction causes outward chest vectors, resulting in chest cavity expansion. As a result there is a decrease in intrathoracic pressure and air flow into the lungs. When the muscles relax, the diaphragm flattens, the intercostals return to baseline position by passive and elastic recoil, and the chest moves inward, causing the chest volume to decrease. The intrathoracic pressure rises and becomes higher than the atmospheric pressure and therefore air will move outward from a position of high pressure to one of low pressure.
Third Layer ? Pleura
The third layer is the double-layered pleura, with one component intimately attached to the chest wall, and the other layer intimately attached to the lung. Between them there is a thin layer of fluid that binds the two layers together by capillary action. The pleural surface constantly absorbs the fluid or gas that enters the space and a negative pressure of about 10mm Hg is maintained. We have all experienced the power of the thin fluid layer of water between two clean smooth surfaces of glass which cannot be pulled apart because of the adhesive and cohesive capillary action. Capillary action is the interaction between contacting surfaces of a liquid and a solid. The cohesion and adhesion that result keep the outer chest cage of bone and muscle in intimate contact with the lungs, being pulled and pushed together in the harmonious dance of respiratory movement.
The adhesion and cohesion of the lung to the chest wall is a lifelong relationship of intimate contact, characterized by 10-15 in and out movements per minute at rest, and sometimes up to 20 – 40 breaths per minute during exercise. The harmony and intimacy continue despite the rigorous jolts of life including violent sneezes, cough whoops, jumps to the basketball hoops and the likes of usual rough and tumble. It is a simple but marvelous mechanism that keeps two structures bound together by such a simple but powerful physical principle. When there is a large effusion or pneumothorax then the cohesive and adhesive mechanisms fail, and collapse of the lung ensues.

Ashley Davidoff, M.D. TheCommonVein.net 42540b06
Fourth Layer – Lung
The lung as a whole provides the next functional layer, but within the lung there is a combination of structures, each with different properties and needs. For the sake of understanding we will consider it a single layer. The relatively rigid upstream airways do not change much with the ins and outs of respiration, while the smaller airways and to greater extent the alveoli undergo significant change in size, expanding with inspiration and getting smaller with expiration.
The alveoli exhibit a surface tension which creates an environment for recoil during expiration and if held unchecked would undergo complete collapse. The tendency for recoil is necessary and healthy, while the collapse is of course harmful. The total collapse of the alveoli is prevented by the automatic rhythm of involuntary inspiration which reverses the process as well as the presence of surfactant (our fifth layer) which prevents the collapse. In the absence of surfactant the pressure required to keep the alveoli open is 20-30mmHg. Surfactant reduces this to about 3-5mmHg.
Fifth Layer – Surfactant
Surfactant, the fifth and last layer, is a lipoprotein solution secreted by specialized cells of the alveolus. It serves to reduce the surface tension and ease the force necessary to open the alveoli during inspiration. In blowing up a balloon, we find that the most difficult part is when the radius of the balloon is small. Thus the surface tension on the balloon is high when the radius is small. As the radius increases the surface tension decreases and it becomes easier to blow up the balloon. Surfactant if it were present in the balloon would decrease the wall tension by an order of 15, to ease the energy and pressure necessary to inflate it.
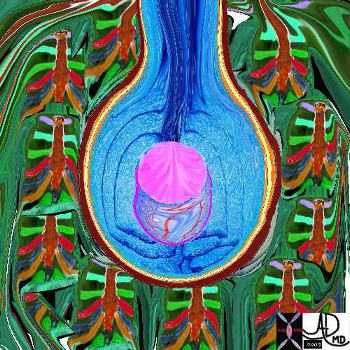
The five major layers that keep the air moving include the outer bony cage, the muscular layer represented in maroon, the pleural complex (orange yellow orange) the lung (blue) and surfactant within the alveolus. (pink)
Ashley Davidoff MD TheCommonVein.net 42530b05b09b01a08

The alveolus is lined by a complex detergent type solution called surfactant (pink) which reduces the surface tension in the alveolus, making it easier for the alveolus to expand during inspiration and preventing alveolar collapse on expiration.
Ashley Davidoff TheCommonVein.net 42530b05b09b01a12
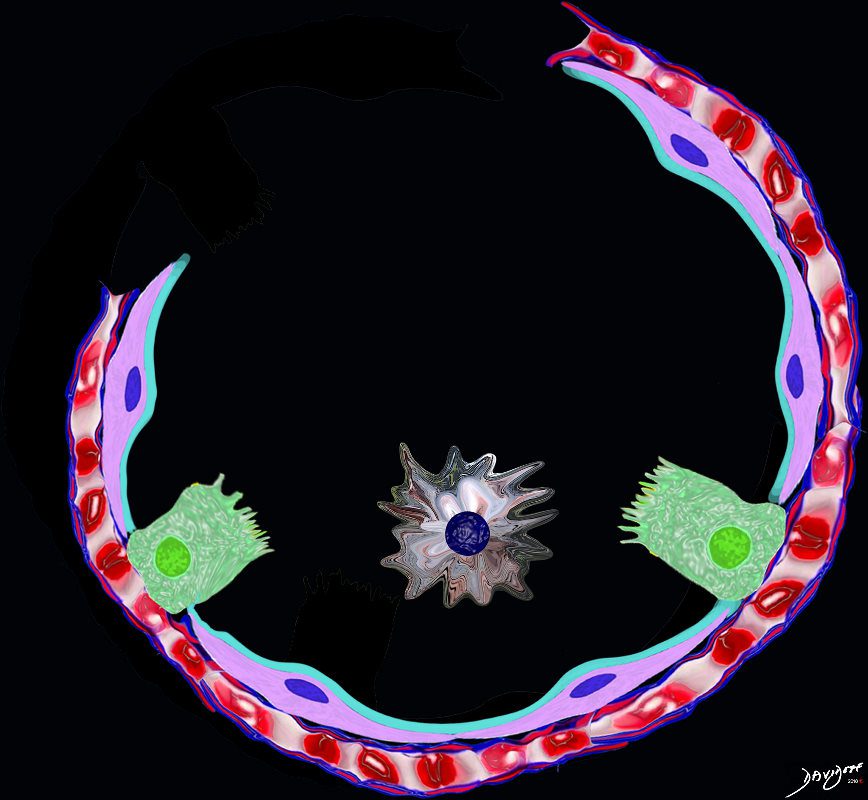
The Buck Ends Here
The alveolus is lined by a simple epithelium ? one cell layer thick. There are two types of lining cells; Type 1 pneumocytes are squamous cells that cover 90% of the surface of the inner lining of the lung , and type II cuboidal pneumocytes that are in fact much more numerous than Type I. They are involved in the production of surfactant . In the lumen there are resident macrophages which play a crucial role in the immune system. The mucosa is grounded by a basement membrane and a lamina propria, and connected to the lamina propria and basement membrane of the surrounding capillary. The alveolus is lined by a thin layer of surfactant. (teal blue)
Ashley Davidoff TheCommonVein.net lungs-00679

This image shows a famous cardiologist and magician, Dr Jonathan Fisher,(with permission) performing as Zippy the clown. Here he reveals and exaggerates the difficulty with surface tension and radius in the early stages of blowing up a balloon.
Ashley Davidoff TheCommonVein.net 60730b01
Lung Movement: Applied Physiology
We will now review the 5 layers and apply our knowledge of the physiology and anatomy to the diagnostic and therapeutic world.
First Layer ? Bone
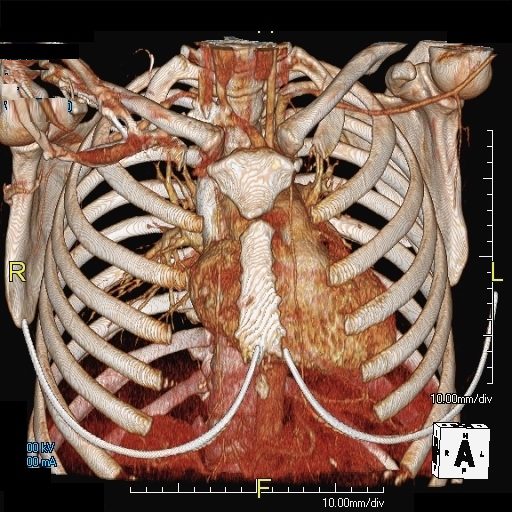
key words bone rib thoracic spine spinous processes scapula protection support
Ashley Davidoff, M.D. TheCommonVein.net
49643
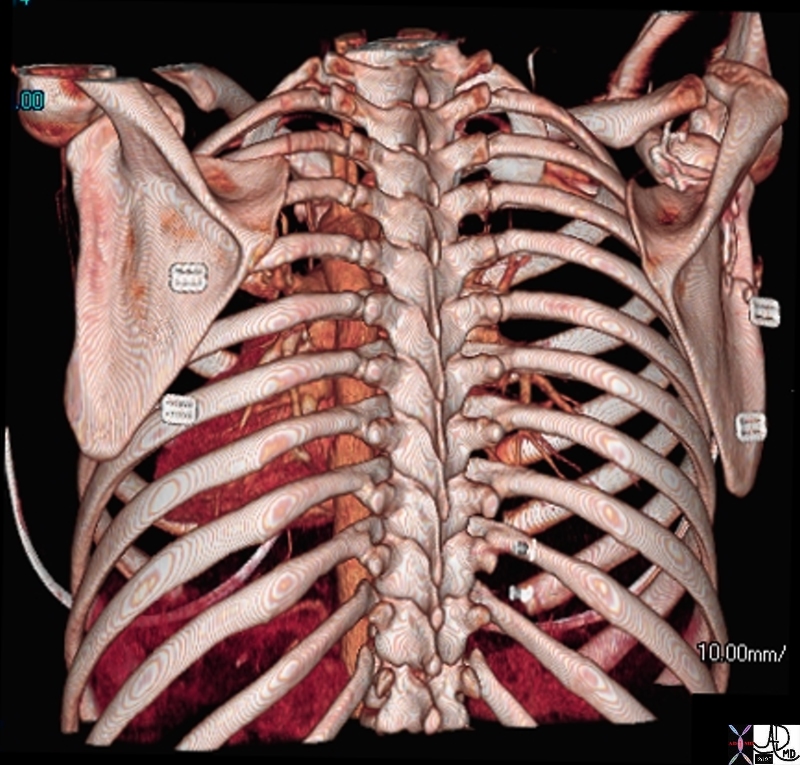
key words bone rib thoracic spine spinous processes scapula protection support
Ashley Davidoff, M.D. TheCommonVein.net
49640
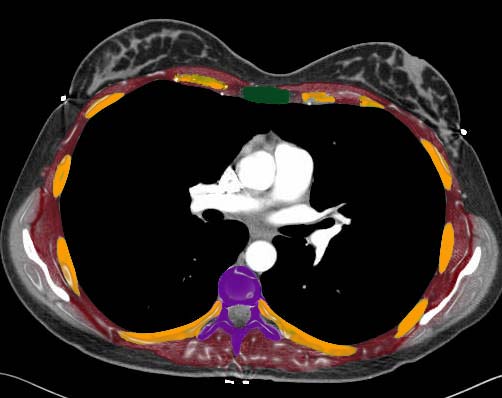
The cross sectional appearance of the outer layer of bone is visualized in this CT scan through the heart. The vertebra (purple) and sternum (green) act as the anchoring stability as they remain fixed in position during the respiratory cycle. The ribs (orange) pivot like bucket handles off the sternum (green) and spinal column (purple). They move up and out during inspiration and down and in during expiration.
Ashley Davidoff, M.D. TheCommonVein.net 42554ab07c
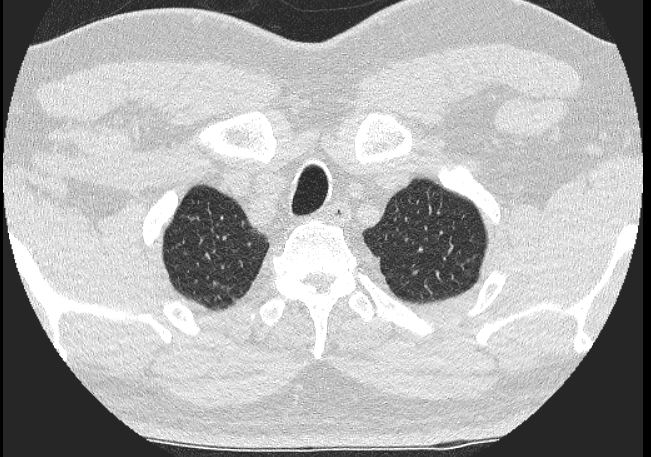
Ashley Davidoff TheCommonVein.net
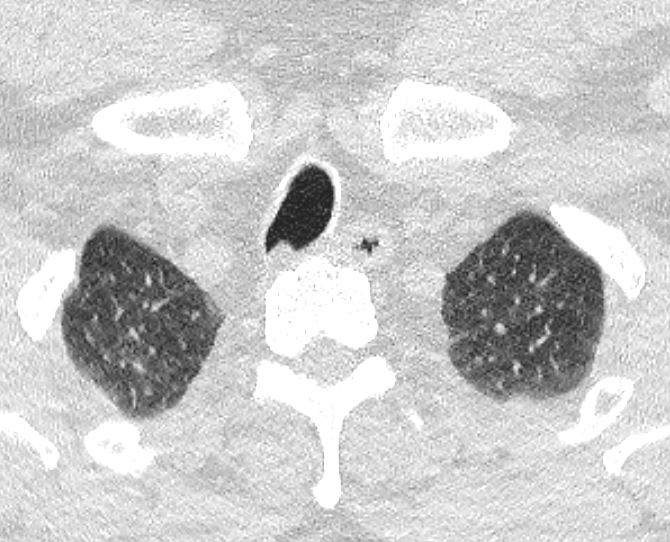
Ashley Davidoff TheCommonVein.net
normal-57-M-00a-inspiration.jpg
InspirationAshley Davidoff TheCommonVein.net
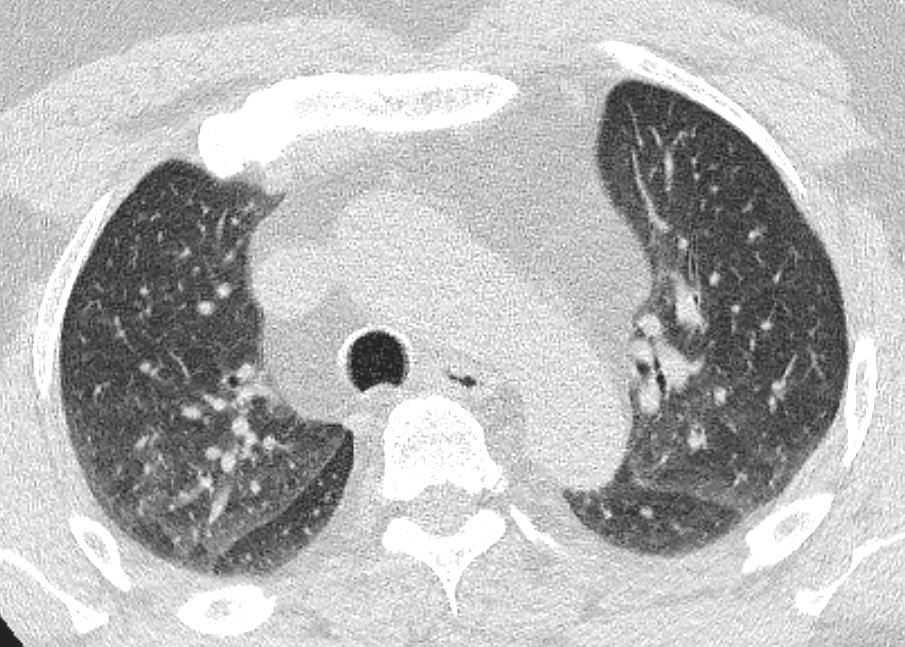
Ashley Davidoff TheCommonVein.net
normal-57-M-00d-inspiration.jpg
InspirationAshley Davidoff TheCommonVein.net

Ashley Davidoff TheCommonVein.net
normal-57-M-00f-inspiration.jpg
InspirationAshley Davidoff TheCommonVein.net
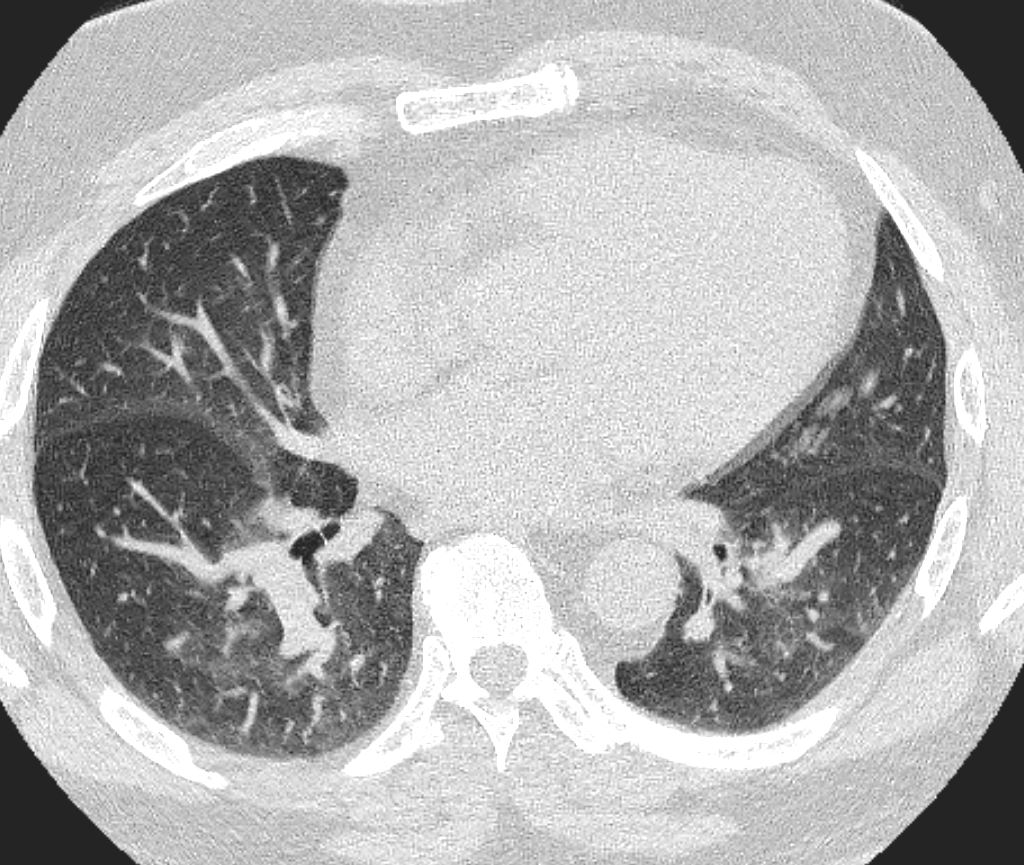
Ashley Davidoff TheCommonVein.net
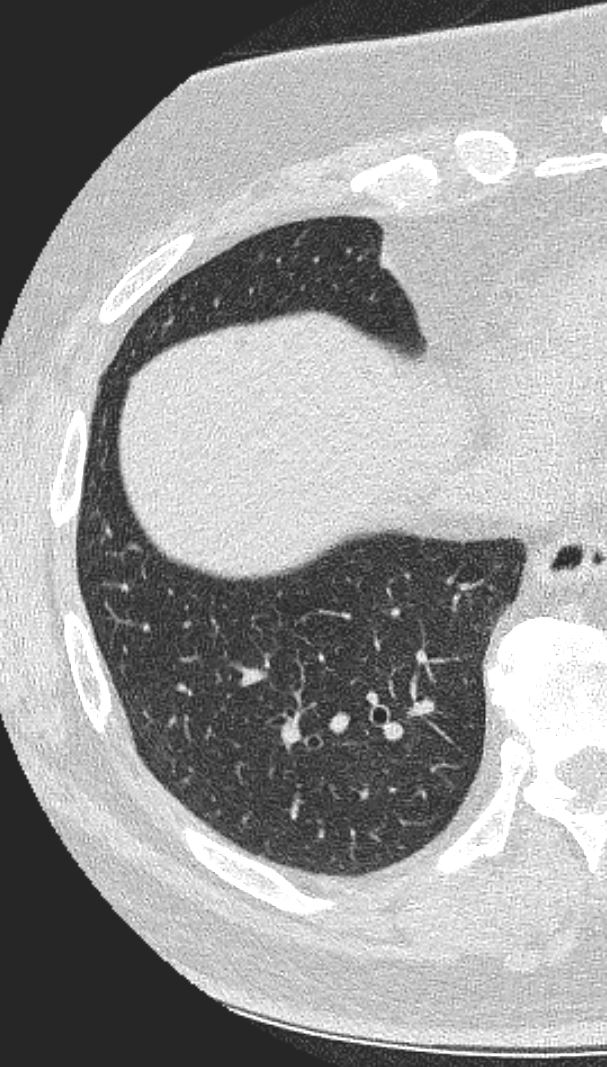
Ashley Davidoff TheCommonVein.net
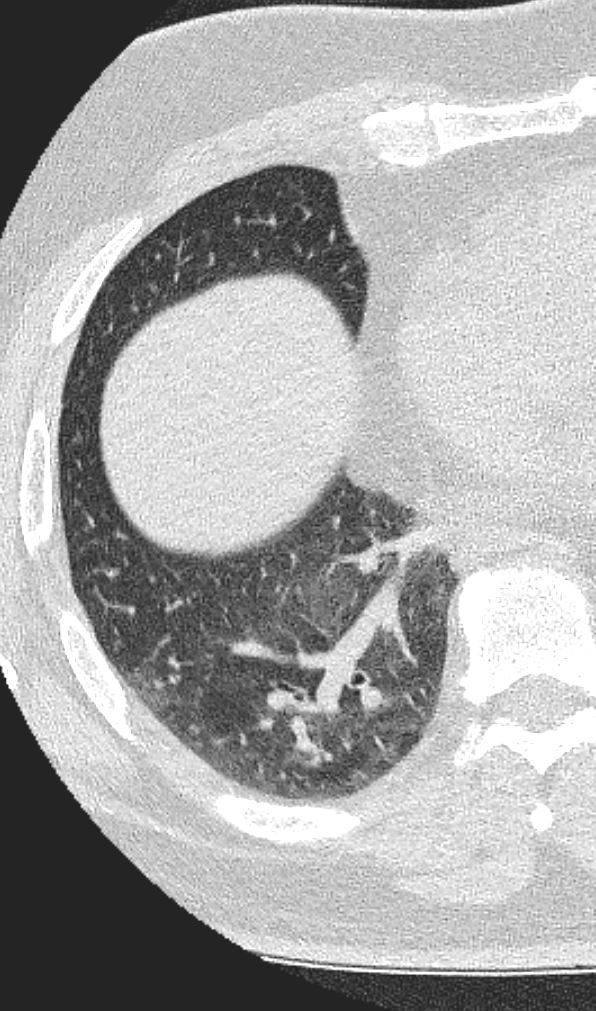
Ashley Davidoff TheCommonVein.net
Fractured Ribs
The fracture of two to three ribs in one place does not result in respiratory difficulty. The pain that ensues can cause decreased respiratory excursion and consequent mild atelectasis. Pain limiting respiratory motion is a very common clinical and radiological scenario, often seen in patients who have had abdominal or chest surgery.
Flail chest, on the other hand, is fortunately not common, but is an urgent clinical problem following extensive blunt traumatic chest injury. Flail chest arises when three or more ribs are fractured both anteriorly and posteriorly, resulting in a loss of chest support. Paradoxical chest movement results so that during inspiration the fractured ribs follow the movement of the lung rather than the chest wall from which they have become detached. Thus during inspiration the isolated ribs and associated chest wall move inward instead of out, and during expiration they move out instead of inward. This injury is usually seen as a consequence of severe trauma, and associated lung parenchymal injury is common. Clinical consequences are a combination of the loss mechanical efficiency and loss of parenchymal function.
The following case represents a controlled situation of paradoxical motion of the chest. The patient was asked to take a deep breath in. Can you work out what the problem is?
The following CT shows the same patient during inspiration.

This patient had to have part of her right chest wall resected including the costochondral complex with its associated muscle. During inspiration there was paradoxical movement of her chest wall with the post op bed moving in while the rest of the chest wall moved out. This is called paradoxical motion since the affected segment is not synchronized with the normal chest and in fact moves in the opposite direction to the normal chest.
Ashley Davidoff, M.D. TheCommonVein.net 42575
The following CT shows the same patient during expiration.
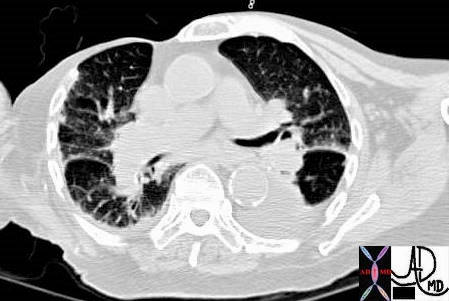
The CT was taken at end expiration. During expiration the chest cage normally recoils moving inward. Because of the lack of support of the chest the affected segment is moving out ward. This is also paradoxical. Courtesy Ashley Davidoff, M.D. TheCommonVein.net 42573
Scoliosis
The effects of scoliosis on respiratory function both before and after surgical correction have revealed that the degree of scoliosis did not correlate with the severity of respiratory difficulty, though there was improvement by about 10% of lung function following surgical correction.
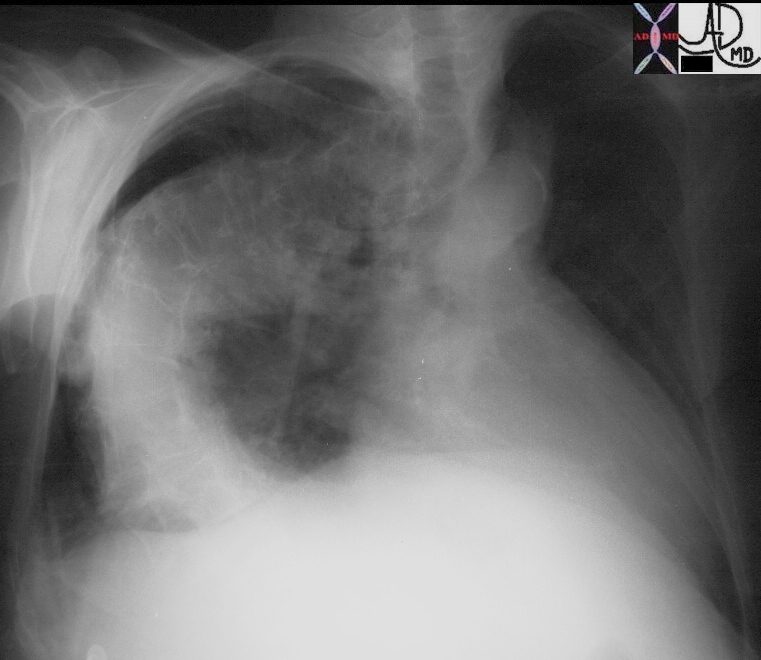
This image of the chest reveals a severe dextroscoliosis of the thoracic spine, The mechanical disadvantage of having a curved support rather than a straight support for lung movement affects respiratory function.
Courtesy Ashley Davidoff, M.D. TheCommonVein.net 29470

The CT of the patient above shows the proximal thoracic spine running at right angles to its normal axis, indicating a severe scoliosis. The anatomy of the lung is severely distorted.
Courtesy Ashley Davidoff, M.D. TheCommonVein.net29476
Second Layer ? Muscle
The ring of muscle is the next layer
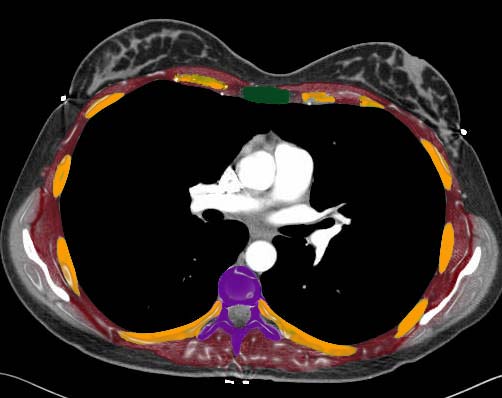
Ashley Davidoff, M.D. TheCommonVein.net 42554ab07c

The diaphragm plays the most important role in breathing. Although it covers a large area it is relatively thin and therefore it usually cannot be fully appreciated on axial cuts. Can you see the diaphragm in this coronal image, and if so why is it so well seen? Additionally why do you think it does not continue all the way across the midline?
The reason we see the diaphragm so well is because there is fluid in both the chest cavity and abdominal cavity and the interface of fluid density on the contrasting soft tissue density of the diaphragm makes it more easily discernible. The central part of the diaphragm is called the central tendon of the diaphragm and it is in continuity with the pericardium. True to its name it is tendinous and therefore very thin and difficult to image.
In this cross sectional image of the same patient the diaphragm is seen as an undulating thin muscle. Since it is dome shaped with its attachments inferiorly only parts in this particular cross section are seemingly attached to the ribs. The undulations are caused by tendinous attachments that extend from the ribs to the central tendon. The full diaphragm was not visualized in this patient and the digitally manipulated image has interpolated the data to provide a circumferential view.
Ashley Davidoff MD TheCommonVein.net 42557b04c
Paralysis
It is not uncommon to see paralysis of one hemidiaphragm without untoward effect. However, when both components of the diaphragm fail, therapeutic intervention is urgent. Diseases that affect the neural signal (polio, Guillain Barre, quadriplegic syndromes), transmission of the neural signal (myasthenia gravis), or disease of the muscle itself (tetanus, myopathy), can result in respiratory failure. In the acute situation when respiratory compromise is severe the patient will require a ventilator. The iron lung was developed to treat polio in 1927. It was the first respirator and was developed by a Harvard researcher Philip Drinker. He used an iron box and two vacuum cleaners to create a push and pull motion on the chest.
Third Layer ? Pleura
The next layer is the pleura which act as the intermediary between the chest wall and the lungs.
The pleural adhesion/cohesion mechanism is quite robust system, but like all mechanical systems under duress it can fail. Pneumothorax is a well known failure of this system and is caused by air leaking from the lung, which intervenes in the bonding mechanism and separates the two pleural components.
Pneumothorax

This case shows a chest x-ray of a patient who had a lung biopsy which was complicated by a pneumothorax and a small amount of pleuroparenchymal hemorrhage. The two components of the pleural layer have been separated and the cohesive/adhesive forces have been disrupted by the air which now intervenes and disturbs the physics of the capillary action. The parietal pleura remains attached to the chest wall while the visceral pleura remains attached to the lung. This is a small pneumothorax and had no effect on the patient or the mechanics of lung movement. In this case we followed the patient with a CXR at 2 and 4 hours later. When no progression was demonstrated we allowed the patient to be discharged home with special instructions to limit activities till the next day. In this case there is an abnormal increase in the density of the visceral pleura
Ashley Davidoff MD TheCommonVein.net 42041b01

The above image is a magnified view of the pneumothorax.
Courtesy Ashley Davidoff MD TheCommonVein.net 42041b02
When a patient has a small pneumothorax we ask that the patient lie in bed with the affected lung on the down side (ipsilateral decubitus position) so that we limit the chest wall movement on that side and hence air movement into the lung. If the pneumothorax is large the patient can become symptomatic and a life threatening event called tension pneumothorax can ensue. In tension pneumothorax, air leaks into the pleural space on inspiration and is trapped on expiration. The air subsequently accumulates untl the pressure is sufficiently high in the pleural space to cause compression of the venous structures in the chest. The elevated venous pressure prevents venous return to the heart and cardiac output falls. Quick recognition of this entity and immediate decompression by a needle or a tube is life saving.
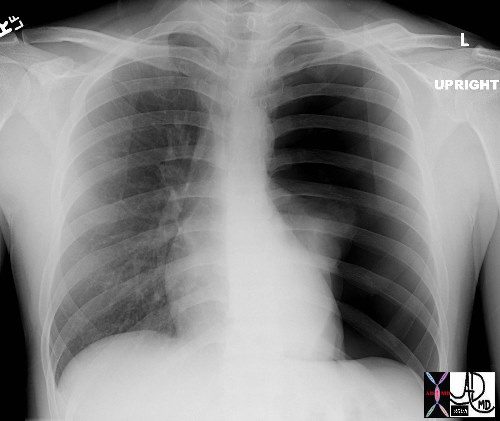
This is the type of CXR that sends shivers down the spine. The overall blackness of the left chest cavity, in association with a nubbin of lung tissue in the ipsilateral hilum and rightward mediastinal shift is characteristic of a tension pneumothorax with total atelectasis of the left lung. Immediate and urgent decompression with a chest drain is indicated.
Courtesy of: Ashley Davidoff, M.D. TheCommonvein.net 42525
Pleural Effusion Problem with Layer 4
The following is also a problem with the pleural space ? Can you work out what the problem is in the right chest?
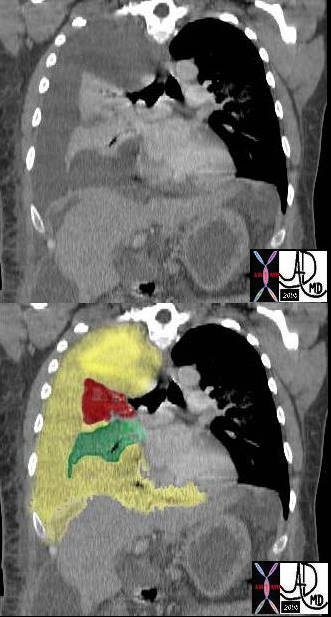
In this case there a large right sided pleural effusion (yellow) with secondary atelectasis of the right lung. (red and green) This coronal CT of the chest at the level of the left ventricle shows a large right pleural effusion which lies between the visceral and parietal pleura. Once the effusion is large enough to weaken the capillary forces that hold the parietal and visceral pleura together, it fail, and the lung collapses which is what is noted on this image ? ie total lung collapse because of loss of cohesive adhesive forces.
Courtesy of: Ashley Davidoff, M.D. TheCommonvein.net 42558c
Fourth Layer ? Lung
Restrictive Lung Disease
The next layer is the lung. Restrictive lung disease occurs when the interstitial tissue of the lung lose elasticity and is replaced by fibrotic tissue. The patient has greater difficulty in expanding the lung and the recoil mechanism is also affected.
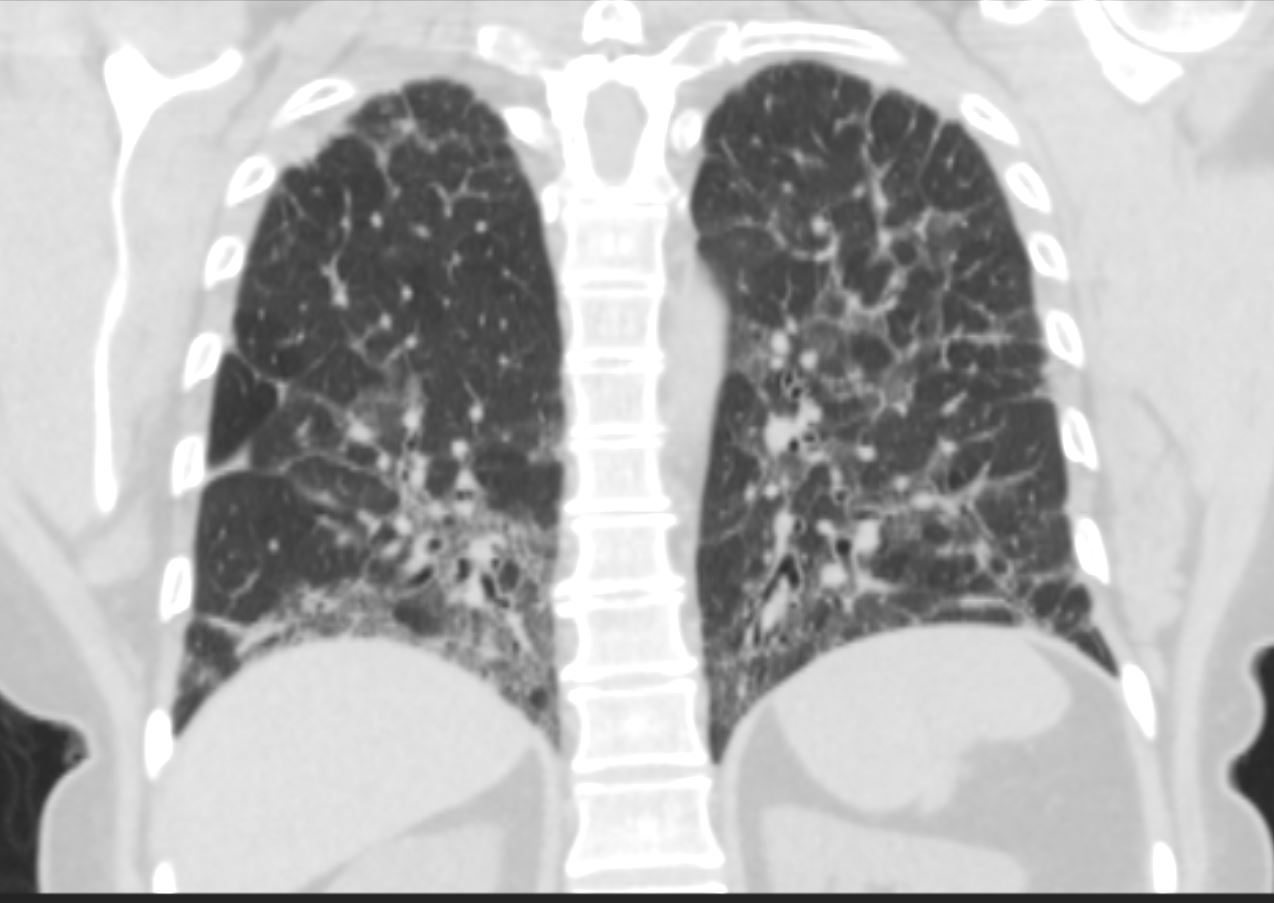
Ashley Davidoff MD TheCommonVein.net 55f ILD
Fifth Layer ? Surfactant
Hyaline Membrane Disease
The next layer is the surfactant.
Premature babies do not produce surfactant and therefore their tendency to collapse the alveoli during inspiration is increased. Also, greater forces are necessary to inflate the alveoli. Extensive atelectasis or lung collapse results in this condition and the baby may require a respirator to help keep the lungs open. This disease is called hyaline membrane disease, or respiratory distress syndrome.

CT scan of a 79year old male at the level of the aortic arch shows diffuse ground glass infiltrates
centrally located with some subpleural sparing
Ashley Davidoff TheCommonVein.net 134294
A parallel disease in older patients is called ARDS, or adult respiratory distress syndrome. It is characterized by diffuse alveolar damage (DAD), resulting in a relative lack of surfactant and consequent micro-atelectasis. There are many causes of DAD including sepsis, multisystem trauma, smoke inhalation, near drowning, and narcotic overdose. The absence of production of surfactant, in combination with the fluid accumulation in the alveoli, causes increase surface tension and more patient effort to expand the alveoli. When this effort fails there is atelectasis that may require a respirator to keep the alveoli patent.
Gas Exchange: Basic Principles
While the function of air transport is the domain of the tracheobronchial tree, it is the function of the alveoli to act as a membrane for gas exchange to and from the atmosphere and the blood. The principles of gas exchange across a membrane are also universal in the body. The movement of both oxygen and carbon dioxide is by diffusion. Diffusion is the movement of the gases, fluids or chemicals from a higher level of concentration to a lower level. The partial pressure of the two gases is a measure of their concentrations. The greater the difference in partial pressure the greater the rate of diffusion. Oxygen is carried mostly by a macromolecule of protein called hemoglobin while carbon dioxide is mostly carried in the form of bicarbonate in the serum.
The membrane across which the gases have to move is between .3 – 1.0 micron thick and consists of two cell layers. One layer is the epithelium of the alveolus and the second layer is the epithelium (called endothelium) of the capillary.
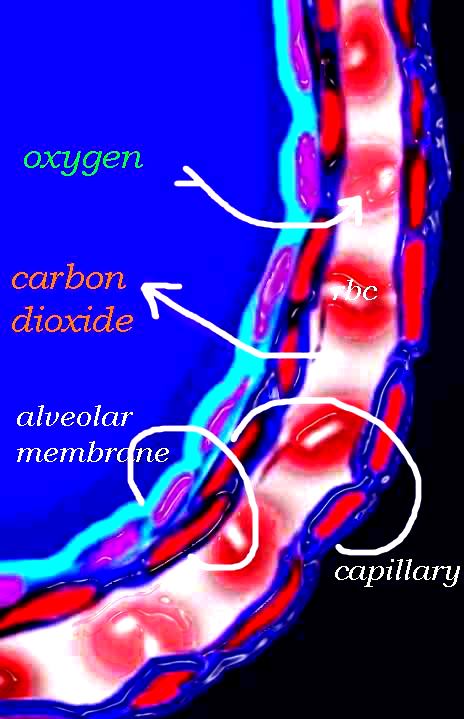
The diagram shows an alveolus, lined by a single layer of squamous cells, surrounded by a capillary with red cells which is also lined by a single layer of squamous endothelial cells . The images show exchanges of oxygen and carbon dioxide through the alveolar membrane .
Ashley Davidoff MD TheCommonVein.net lungs-0028b-low res
Fick?s law governs the movement of air across a membrane. The net movement or diffusion rate of a gas across a fluid membrane is proportional to the difference in partial pressure, proportional to the area of the membrane and inversely proportional to the thickness of the membrane. The total membrane surface area of the alveoli is about 80-100 square meters and the thickness is less than a millionth of a meter, so it is a very effective gas exchange interface.
At rest about 6-10 liters of air is moved through the tracheobronchial tree per minute. In this same minute about 600ccs of oxygen moves from the alveoli into the blood, while 600ccs of carbon dioxide moves across the membrane into the alveoli. During exercise up to 100 liters of air can be moved through the airways per minute and about 3 liters of oxygen and 3 liters of carbon dioxide is moved across the membrane.
Composition of Air
Dry air is composed of 21% oxygen, .04% carbon dioxide, 78% nitrogen and less than 1% of other gases such as argon and helium. The amount of gas present can also be expressed in terms of its partial pressure. At sea level the partial pressure of oxygen is 160mmhg (21% of 760mmHg) and carbon dioxide is .3mmhg in the atmosphere. In the lung the partial pressure of carbon dioxide (pCO2) is relatively higher being 40mmHg and the PO2 is 104mmHg, while the PCO2 in the pulmonary arteriole is 45 and the PO2 is 40mmHg.
Changes in PO2
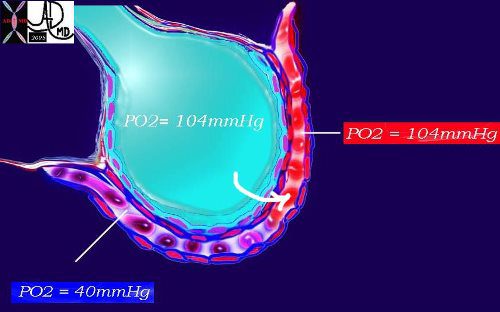
This diagram again shows the alveolus in teal, the arteriolar component of the capillary with red cells in blue and venular component replenished by oxygen in red. As noted above, the PO2 of the arterial blood is 40mmHg while the inspired air is 104mmHg. A pressure gradient thus exists and diffusion from the high to the low pressure occurs with a net movement of oxygen into the blood to equilibrate the pressure. Venous blood is now rich in oxygen with a PO2 of 104mmHg.
Ashley Davidoff MD TheCommonVein.net 42445b08b
Changes in PCO2

This diagram shows the PCO2 of the arterial blood at 45mmHg with red cell again showing telling signs of blue deoxygenation while the inspired air has a PCO2 of 40mmHg. There is therefore diffusion from the high to the low pressure and a net movement of carbon dioxide into the alveolus to equilibrate the pressure of 40mmHg. The venous blood is thus relatively depleted of CO2 with a PCO2 of 40mmHg. |
Ashley Davidoff MD TheCommonVein.net 42445b11
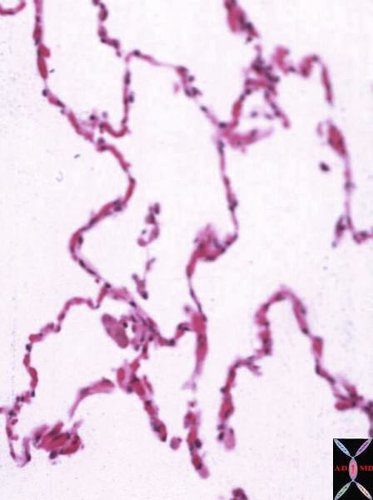
Lower magnification of the lung with H and E stain shows cup-shaped alveolar spaces outlined by delicate thin alveolar capillary membrane.
key words
lung, pulmonary, normal alveolus, alveoli, histology, interstitium, interstitial
Courtesy Armando Fraire MD. TheCommonVein.net 32819
Gas Exchange: Applied
The alveolar membrane can get affected by diseases processes that may accumulate fluid or pus within the alveoli causing a thickened membrane. Interstitial fibrosis is a second cause of thickening of the respiratory membrane which will affect the efficiency and speed of gas exchange.
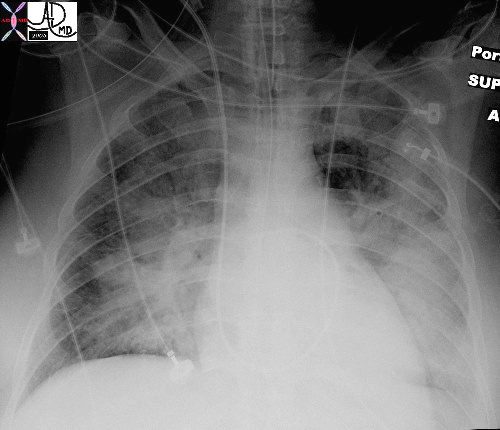
In this patient with acute congestive cardiac failure the consolidation that has hilar distribution has reminded radiologists of bat wings and is caused by alveolar edema. As a result of the fluid in the alveoli, gas exchange across the respiratory membrane is reduced and required intubation to improve the gas exchange process. Note the endotracheal tube as well as the central venous line that is used to assess the heart pressure and monitor the congestion.
Ashley Davidoff MDTheCommonVein.net 42073b01
In general we intuitively know that when we take a deep breath we are using our muscles to actively inspire. When we breathe out it is a relaxation of that mechanism caused by actual muscle relaxation and the elastic recoil of the layers of the chest. We can of course breath out all the way until we have expired right to what we think is the last drop of air, and this takes effort and muscle contraction as well. When you as a technologist have a patient for a routine CXR you ask your patient to ?take a deep breath in, blow it all the way out and hold it?.? And you click your exposure. You can readily see that there is still quite a lot of air left in the lung. This is called the dead space of the lung, found in patients who are well and alive! Read on and learn all about what happens to the lung volumes and capacities during that process.
Lung Volumes
In order to explain the process we will pretend that you are a lung function technologist for a moment. You are going to test your patient?s lung volumes, capacities and velocities. We want you to think and apply what you see on these graphs to your own situation when you are doing a CXR, and to imagine the differences between an inspiration CXR and expiration CXR. We particularly want you to see the difference in volume of air between the inspiration film and the expiration film.
So we go back to our theoretical patient undergoing lung function tests and we place a mouthpiece over her mouth and attach the tubing on the other end to a recording device called a spirometer. As our patient lies on the table breathing quietly under resting conditions we notice a simple rhythmic pattern on the spirogram. This pattern is entirely involuntary caused by the production of a signal from center of respiration located in the brain that causes the diaphragm and other muscles to contract and to expand the chest, and then as it switches off, the muscular relaxation and elastic recoil cause the chest to contract.
Tidal Volume

Courtesy of: Ashley Davidoff, M.D.
TheCommonVein.net
42529b06b01b
We reset our spirometer to get our basal conditions set between the 3 and 4 liter mark so that we have room on the graph to see what happens with forced inspiratory effort and forced expiratory effort.
For those of you who require your patient to ?breath hold? for MRI and CT, we are in fact asking them to stop this involuntary breathing pattern in order to prevent chest wall and lung movement that would otherwise cause motion artifact on our images.
For our CXR we now ask our patient to take as deep a breath in as possible and we see a dramatic change on the graph.
Inspiratory Reserve Volume

Courtesy of: Ashley Davidoff, M.D. TheCommonVein.net 42529b06b02
When we ask your patient to take a deep breath in we have a similar experience but the change is reflected in a CXR is a larger chest volume by more than 3 liters. The patient will muster their muscular forces to contract the diaphragm and intercostal muscles to enlarge the chest cavity and pull in air. The lung in the healthy patient will now contain about 6.7 liters of air. When our patients can take a deep breath and hold it in, the radiologists are better able to identify disease processes since the interface of air and the soft tissue of the disease is larger and therefore the disease is more easily seen.
For those of you who are ultrasound technologists you use the consequences ?deep breath in? to bring the diaphragm and upper abdominal organs down, beyond the rib cage, in order to optimize your window to the upper abdomen.
Now as the patient reaches peak inspiration we tell her to breath out as completely and rapidly as possible, and our spirometer reading does the following;
Forced Expiration
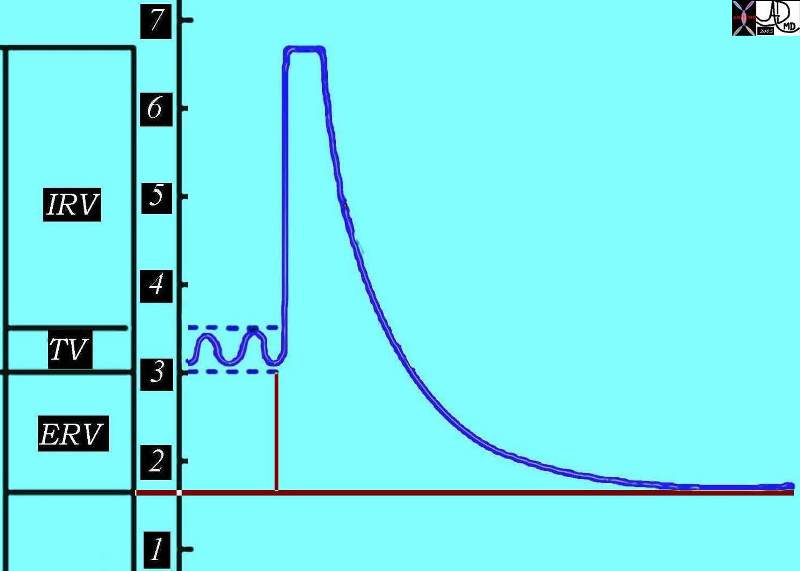
Courtesy of: Ashley Davidoff, M.D. TheCommonVein.net 42529b06aa02
The difference between the end of basal expiration and complete expiration is called the expiratory reserve volume. (ERV)
For the lung function technologist the speed with which the expiration is performed is a key issue in lung function, but for you the degree to which the patient can empty their lungs is key for a good expiration film.
At the end of this complete expiration, there is still a volume of air left in the lungs and airways, and this is called the residual volume (RV), which is the air filled space that keeps everything open after a full expiration. When we perform a CXR in expiration, we ask our patients to breathe in and then all the way out, and to hold it out, and we then expose our film. The air you see in the lungs at the end of expiration is called the residual volume. The lungs are at their smallest volume in end expiration and at this point contain about 1.5-1.7 liters in our patient. In the normal adult the RV ranges between 1.0 and 2.4 liters.
Residual volume
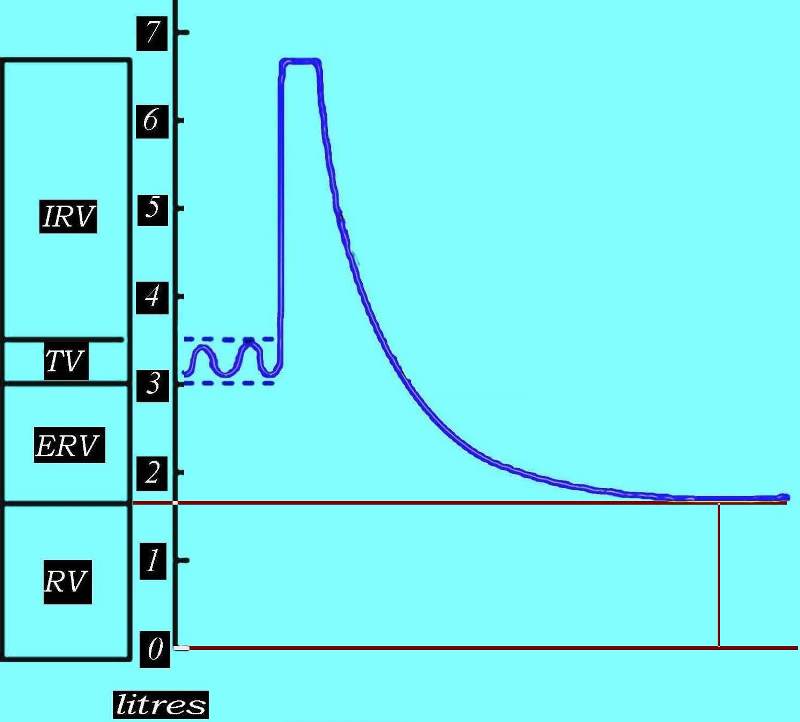
This diagram outlines the residual volume between the red lines, representing the air that is left in the airways and the alveoli after a full expiration. The air you may see on expiration CXR is the residual volume and as you can see it is about 1.8 liters in this patient.
Courtesy of: Ashley Davidoff, M.D. TheCommonVein.net
42529b06aa03
Lung Capacities
We now move on to our patient?s lung capacities, which represent a combination of the volumes we have spoken about. What do you think inspiratory capacity is? It is of course the combination of the basal inspiration which is our tidal volume of 500css combined with the inspiratory reserve volume (3.2liters) giving us a total of a 3.7 liters capacity for inspiration
Inspiratory Capacity
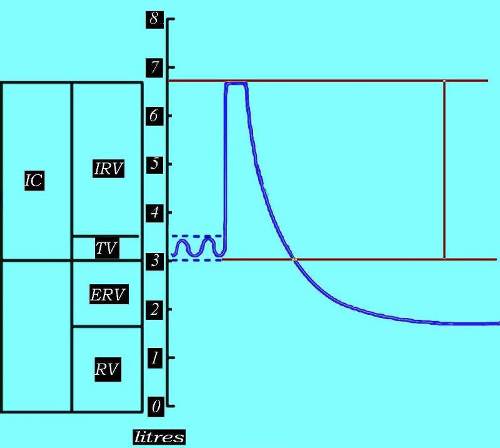
The inspiratory capacity (IC) is the total volume that the person can inspire, and is a combination of the inspiratory component of the tidal volume and the inspiratory reserve volume.
Courtesy of: Ashley Davidoff, M.D. TheCommonVein.net 2529b06aa04
Functional Reserve Capacity (FRC).
The amount of air left in the lungs after a resting expiration includes the expiratory reserve volume and the residual volume is called the functional reserve capacity (FRC).

The combination of ERV and RV is called the functional residual capacity (FRC) as marked by the vertical red line
Ashley Davidoff, M.D. TheCommonVein.net 42529b06aa05d
The vital capacity (VC) is defined as is the maximum volume of air expelled after a maximum inspiration. For you, this is the difference in amount of air in the lungs between a CXR with maximum inspiration and a CXR with maximum expiration. In this patient it is about 5 liters ? a large difference in volume and a large difference in the appearance of the chest, sometimes making the difference as to whether a pneumothorax is seen or not seen. Why is this so important? A small pneumothorax itself may not have any functional significance meaning the patient has no untoward effect. It does however reflect a leak, and a leak can get better or worse. In the situation in which it gets bigger it can cause a life threatening situation and so it is essential to make the diagnosis of even a small pneumothorax. Using expiration technique for the clinical question of pneumothorax is essential. .
Vital Capacity (VC)
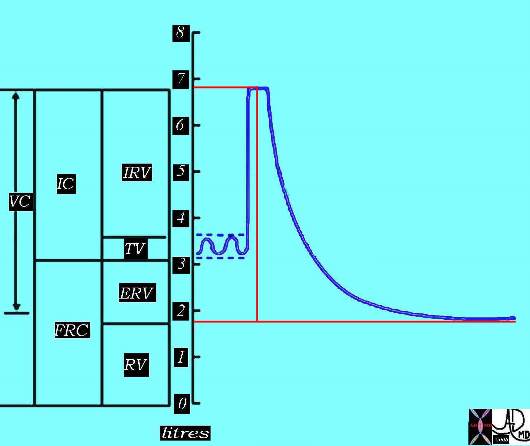
The vital capacity is the maximum volume of air expelled after a maximum inspiration. It also can be viewed as the maximum amount of air that the patient can move in and out of his or her lungs.
Courtesy of: Ashley Davidoff, M.D. TheCommonVein.net 42529b06aa06bd
Total Lung Capacity
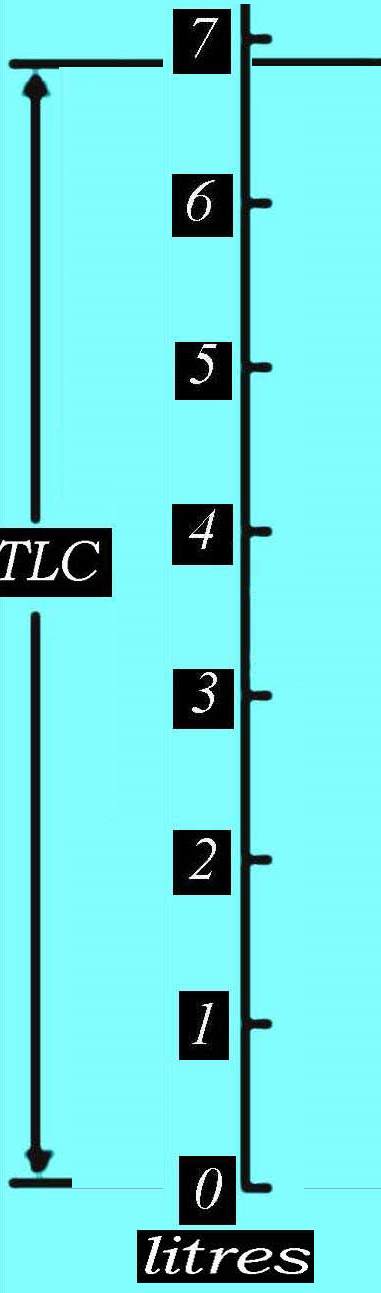
The total amount of air in the lungs after a deep inspiration is called the total lung capacity and is a combination of the vital capacity VC and the residual volume. Thus it is a combination of the maximum volume of air that the patient can move in and out of his lungs and the air that remains in the lungs after a forced expiration. In our patient it is about 6.8 liters. Courtesy of: Ashley Davidoff, M.D. TheCommonVein.net 42529b06aa
Total Lung Capacity

The total lung capacity (TLC) is the total amount of air in the lungs and airways after a deep inspiration. In our patient it is about 6.8 liters.
Ashley Davidoff, M.D. TheCommonVein.net 42529b06aa07
Ventilation and Perfusion
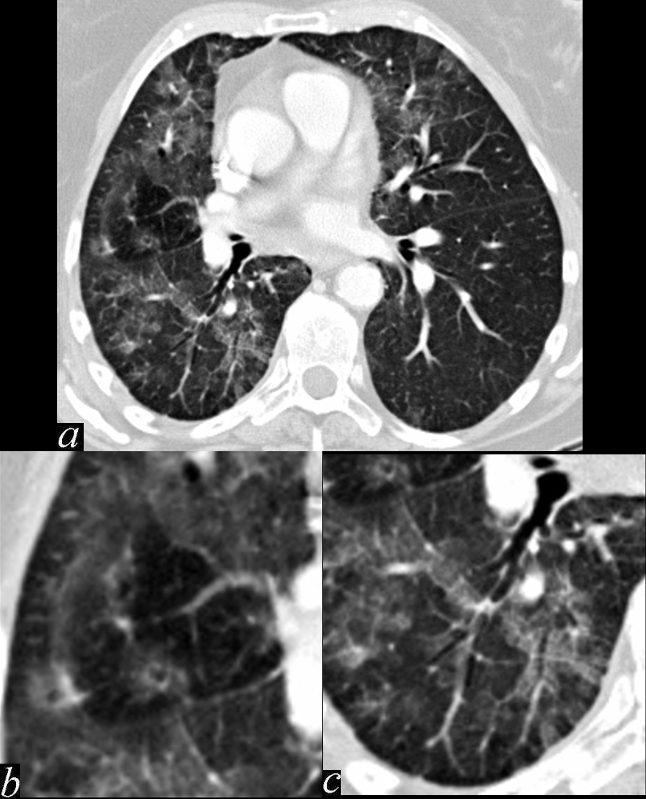
This series of images shows some subtle changes that reflect the local control of blood flow to a small segment of the right middle lobe. Note that in image a, there is a small area of increased lucency (blacker) in the right lung just lateral to the vessels of the right hilum. This region is highlighted in b. Note also that in b, the rapid diminution of the size of the blood vessel to that subsegment when compared to the size change of the vessels in the image in c. The lucent appearance of the lung suggests air trapping and the vasoconstriction reflects decreased perfusion ? ie with decreased ventilation there is an associated consequent associated decrease in perfusion.
Keywords # broncho-centric inflammation lung bronchovascular bundle chest inflammation peribronchial halo air trapping mosaic perfusion ground glass changes alveolar change air bronchogram acute bronchovascular inflammation ddx allergic collagen vascular disease infection CTscan
Ashley Davidoff MD TheCommonVein.net 47170c01.800